CHAPTER 5: Dynamics of the Ecosystem
The ecosphere can be viewed as a large machine comprised of various cycles and systems. Energy required to drive these cycles is predominantly provided by the sun. The environment is therefore not a static entity; rather, it continually rejuvenates and sustains itself through energy flow and the cycling, or renewal, of all matter.
Water undergoes recycling from the atmosphere through the soil and biotic components in what is known as the hydrological cycle. Gases such as oxygen and carbon dioxide are consistently circulated through ecosystems. Even soil, along with its minerals, participates in a continuous cycle known as the sedimentary cycle. Collectively, this is referred to as biogeochemical circulation.
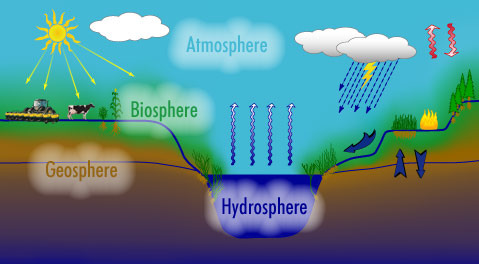
Figure 5.1: Energy required to run cycles in the ecosystem (soil, water and organisms) is provided by the sun.
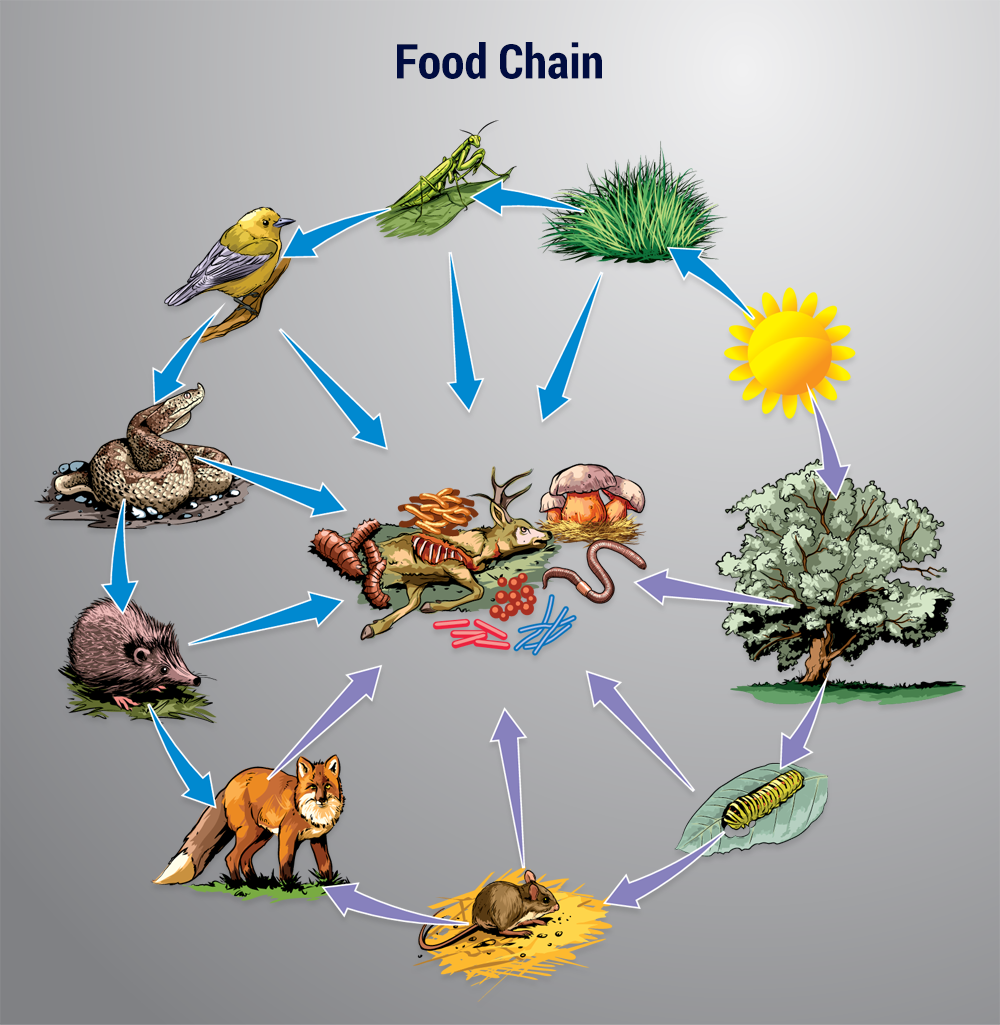
Figure 5.2: Energy is supplied by the sun – used within the ecosystem – and passes out of it. mostly in the form of heat.
The sun acts as the principal source of light and energy, allowing vegetation to generate chemical energy (food) for all organisms, including decomposers. This cycle continues until it concludes in death and decomposition, releasing organic matter back into the system for absorption by plant roots.
It has been demonstrated that organisms are consistently consumed by those positioned at higher levels in a food web (Fig 4.4). Consequently, each level of a food web contains less energy than the one below it. The flow of energy and matter through ecosystems is depicted diagrammatically in food chains, food webs, and ecological pyramids (Fig 4.7). With each transfer of nutrients from one component or level to another, a significant amount of energy (up to 90%) is lost.
As illustrated, plants constitute the first, or bottom, trophic level, designated as T1 (also known as autotrophs). Herbivores, such as antelope, comprise the second trophic level (T2), while carnivores like lions form the third trophic level (T3).
Top carnivores such as birds of prey occupy the fourth trophic level (T4), while T5 (tertiary carnivores) is represented by decomposing organisms in the soil, which return nutrients to the above-ground circulation. Organisms from T2 to T5 are referred to as heterotrophs. These organisms cannot produce their own food and must obtain nutrients and energy from organic compounds produced by other organisms. In contrast autotrophs produce their own food through processes like photosynthesis in plants.
The classification into trophic levels is not based on specific species, but rather on the functions fulfilled by species within the ecosystem community. Trophic levels denote the position of organisms within the food chain, whereas food webs delineate the composition of ecosystems. Each trophic level may contain numerous individuals and diverse populations, all interconnected by the flow of energy (food). Various types of ecological pyramids can illustrate the role of organic matter in ecosystems.
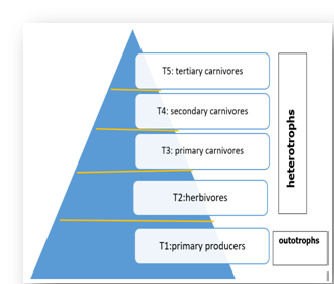
Figure 5.3: The division of trophic levels is based on the function that a species fill in the ecosystem-community.
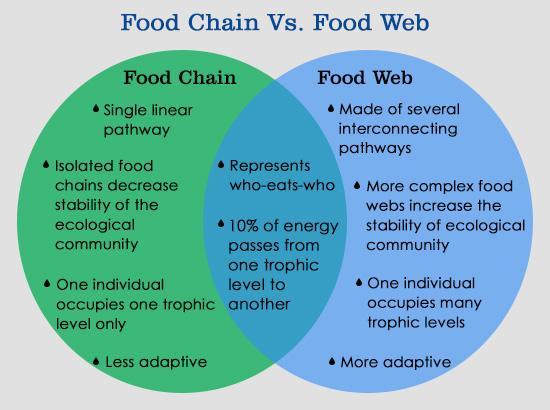
Figure 5.4: Both food chains and food webs are important concepts in ecology because they help scientists understand the dynamics of energy flow and the relationships between different organisms in an ecosystem.
In a numbers pyramid the number of organisms in each trophic level is considered without regard to the size of the organisms. Under typical conditions, there are more individuals at T1 than at T2, and notably fewer at T3, and so forth. This results in a pyramid shape with a broad base tapering towards the top, as depicted in Figure 5.5 (Grassland ecosystem and Pond ecosystem). However, this ecological pyramid might excessively highlight the significance of numerous small organisms, such as lice on a plant, as illustrated in Figure 5.5 (Forest ecosystem or Parasitic food chain on a single tree).
In the biomass pyramid (Figure 5.6), the total relative mass of organisms in each trophic level decreases as one ascends within the system. The specific kilograms can vary depending on the ecosystem. In an energy pyramid (Figure 5.6), the total amount of energy present in each trophic level is displayed.
Thus, it will also depict the decline in energy as it moves from one trophic level to the next. This demonstrates the evident decrease in energy transfer between successive trophic levels.
Consequently, the energy pyramid serves as a more prevalent tool for comparing diverse ecosystems. The diagram exemplifies the “Rule of 10” mentioned earlier, signifying that merely 10 percent of energy is transferred between consecutive trophic levels.
For instance, a bird consuming a catepillar will only receive 10% of the energy obtained by the catepillar from the leaves it consumed.
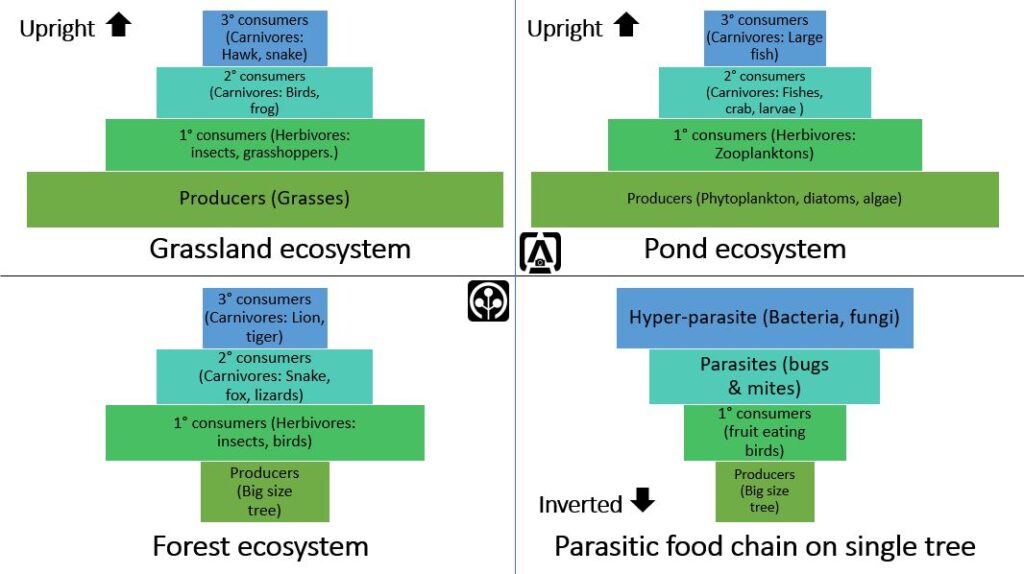
Figure 5.5: The portrayal of trophic levels varies based on the numbers of individual organisms present at each level, will appear differently across various circumstances.
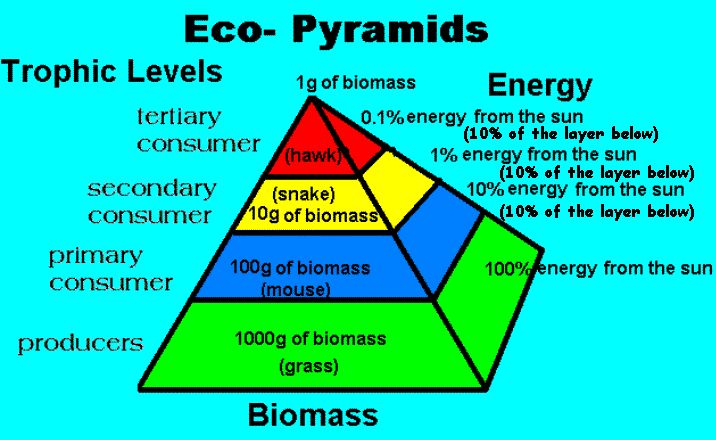
Figure 5.6: A biomass pyramid indicates the combined mass of all individuals on a particular trophic level. An energy pyramid indicates the combined energy present at each trophic level.
The delineation of trophic levels is not always straightforward, as organisms frequently consume food from multiple levels. For instance, certain carnivores also ingest plant matter, and some plants exhibit carnivorous behavior.
Additionally, a large carnivore might prey upon both smaller herbivores and carnivores, while animals can engage in cannibalism, such as when bullfrogs consume crayfish, which in turn prey on young bullfrogs.
Moreover, the feeding preferences of a juvenile animal, and thus its trophic level, can evolve as it matures.
It is crucial to recognize that there is a constant flow of matter and energy within ecosystems.
We will now examine the primary cycles within the ecosystem. Each of these cycles relies on a fundamental reservoir, where elements or compounds are stored for varying durations before reintegrating into the cycle. In the hydrological cycle, for instance, the ocean serves as the primary reservoir. The atmosphere functions as the reservoir for gaseous cycles, while the Earth’s crust acts as the reservoir for sedimentary or soil cycles. Additionally, there are exchange pools where elements or compounds are temporarily held. Clouds serve as exchange pools in the hydrological cycle, and organisms’ bodies function as exchange pools for various chemicals within the biotic community. These cycles, which transport chemicals essential for life across both the biological and geological realms, are known as biogeochemical cycles.
The water cycle, also known as the hydrological cycle, can be simplified as follows: water evaporates from the surface of the oceans, forming moisture that is transported by wind over landmasses.
There, it condenses to form clouds and precipitates as rain, hail, or snow. Some of this water infiltrates into the ground, while approximately 70% returns to the atmosphere as vapor through evaporation and transpiration from plant leaves. The remaining portion ultimately flows back to the sea via rivers and underground aquifers, completing a continuous cycle.
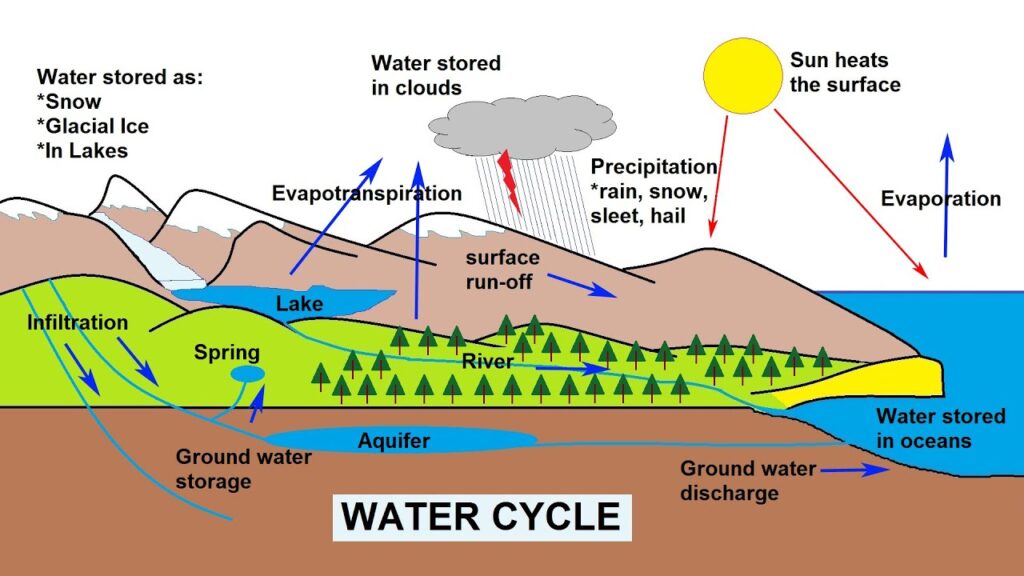
Figure 5.6: Clean water is vital to maintain all forms of life.
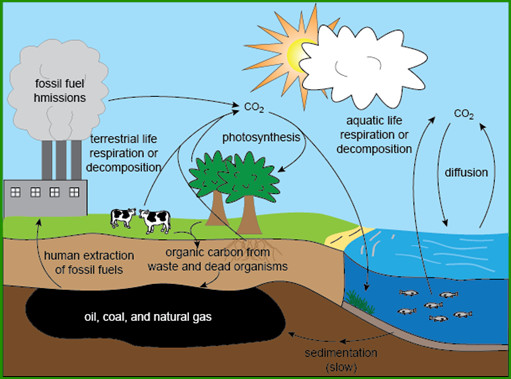
Figure 5.7: Carbon forms the basic building block of all organic compounds
In the colder regions of the Earth, such as the North and South Poles, water can remain trapped for extended periods in the form of snow or ice.
Additionally, water can be temporarily held in lakes, ponds, and wetlands. As water moves towards the oceans, it carries minerals due to the weathering of rocks and eroded soil, contributing to sedimentary circulation.
Organisms also play a crucial role in the water cycle, as up to 90% of their body weight is comprised of water.
Water is essential for many vital bodily functions in humans and animals. Without it, plants cannot uptake and transport chemicals or produce energy in the form of carbohydrates for herbivores. They also cannot release their waste products, namely carbon dioxide and evaporated water.
The quality of water resources, collected in ‘temporary pools’ such as dams and wetlands, and even the sea, are being threatened by various forms of pollution at an extraordinary rate. Water collected from evaporated molecules that returns to the earth in the various forms of precipitation used to be clean from all impurities, but even this is no longer the case as even this ‘cleansing method’ of nature has been affected by air pollution, resulting in acidic rain (Fig. 2.5).
The carbon dioxide cycle can be discussed as an example of the gaseous cycles. Carbon is extremely important for life on earth as it forms the basic building block of all organic compounds, together with hydrogen. The main reservoirs for carbon dioxide are the oceans.
The natural carbon cycle can be seen as a nearly perfect cycle because under natural conditions almost as much carbon is set free through the process of respiration as is bound by photosynthesis. The interference of humans with this cycle is of crucial importance. Due to industrialization, the burning of fossil fuels release enormous amounts of carbon dioxide in the atmosphere. This causes the so-called “Greenhouse” effect leading to “Global warming”.
Green plants, whether on land or in water, absorb carbon dioxide and convert it into carbohydrates through photosynthesis.
Once in the plant, carbon can take three potential paths: it may be released into the air via respiration, remain stored in the plant until it dies, or be consumed by animals feeding on the plant.
When a plant or animal dies, its carbon can undergo two processes: it may be respired into the air as decomposers break down dead material, or it can remain intact and eventually transform into coal, oil, or natural gas.
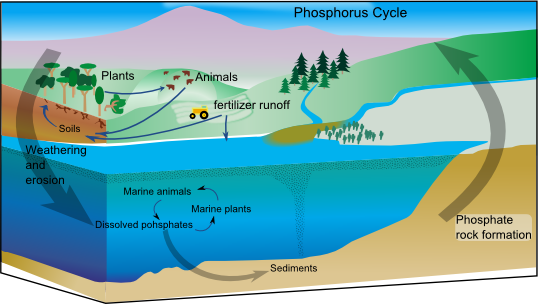
Figure 5.7: Carbon forms the basic building block of all organic compounds
These natural fossil fuel resources may be pushed to the surface by geological forces within the Earth and released through volcanic activity. However, when we artificially expose fossil fuel resources to the environment through extraction and burning, significant amounts of carbon dioxide are emitted into the atmosphere. This contributes to a detrimental effect on the natural environment. Essentially, global warming occurs due to an excess of carbon dioxide in the atmosphere, which leads to more heat from the sun being trapped on Earth rather than escaping into space.
As more carbon dioxide is released into the atmosphere, more carbon is also absorbed by the oceans, leading to consequences such as coral bleaching. As the loss of forests, essential for terrestrial carbon cycling, exacerbates this issue, so similarly, the decline of tropical coral reefs has significant negative impacts on oceanic food chains and ecosystems.
The phosphorus cycle serves as a significant illustration of a sedimentary cycle. When examining sedimentary cycles, it’s crucial to recognize that they comprise two phases: the aqueous solution and the rock phase.Due to its weight, heavy phosphorus molecules do not ascend into the air. Instead, they are consistently found within organisms, dissolved in water, or present in rock formations. When a phosphorus-containing rock is subjected to water, particularly if the water is slightly acidic, the rock weathers and dissolves into the water. Plants rooted in such rock or soil absorb the phosphate, utilizing it, for instance, to construct their cell membranes. Animals that consume these plants incorporate phosphorus into essential structures such as bones, teeth, and shells.
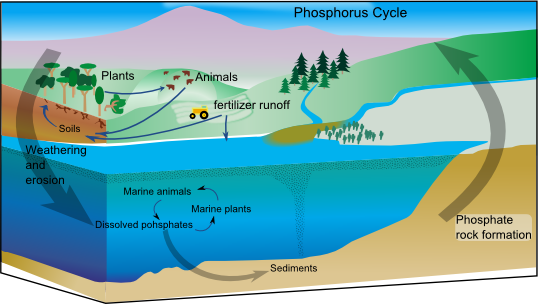
Figure 5.8: An effective phosphorus cycle is crucial, as phosphate serves as a vital component in bones, teeth, and shells and cell membranes.
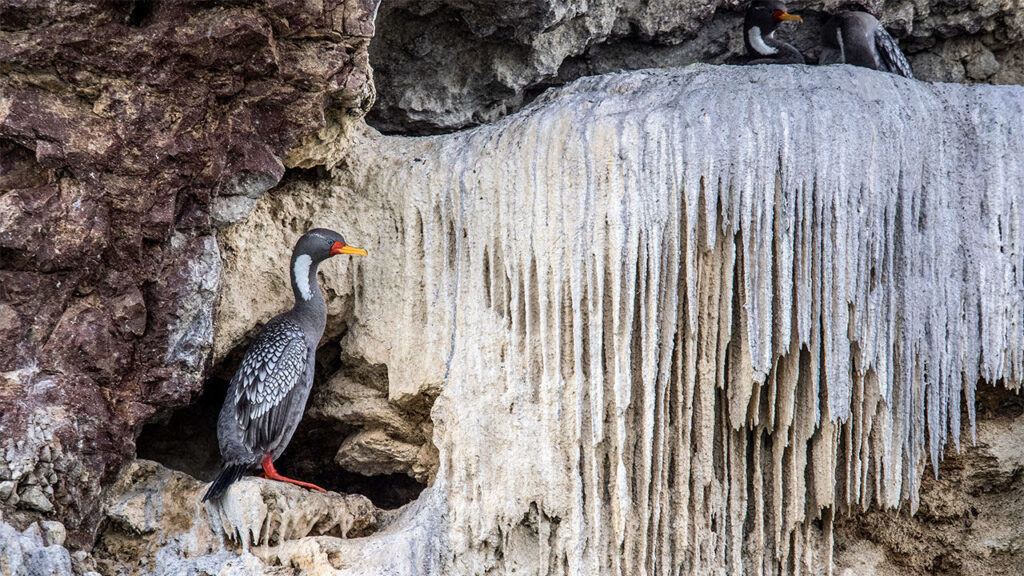
Figure 9: Birds play a significant role in transferring phosphorus from the ocean to the land. The excessive discharge of phosphorus-rich fertilizers and sewage has imposed a burden on natural water systems, leading to eutrophication.
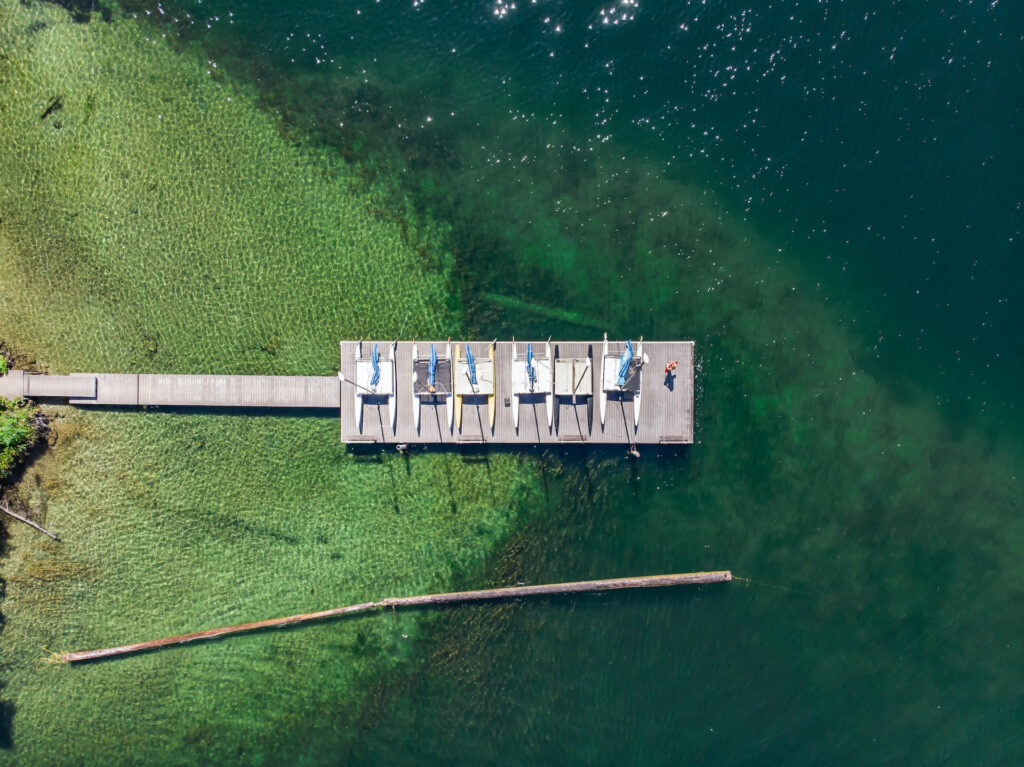
When an animal or plant excretes waste or dies, phosphorus is returned to the soil or water as decomposers break down the remains into a form usable by other plants. This cycle repeats until phosphorus settles on the ocean floor, becoming part of sedimentary rocks formed over millions of years. Eventually, through processes like crustal movement, these rocks may be brought to the surface, exposed to weathering.
Marine birds play a crucial role in returning phosphorus from the ocean to land. Phosphorus enters their systems through fish bones they consume, and the accumulation of their waste is known as guano. Historically, people extracted phosphate from guano or from areas once underwater for use as fertilizer. However, this has led to an overabundance of phosphate in natural water systems due to runoff from agricultural land, resulting in eutrophication. This process causes oxygen depletion in the water due to increased algae growth, leading to the demise of other aquatic life unable to survive in oxygen-deprived conditions.
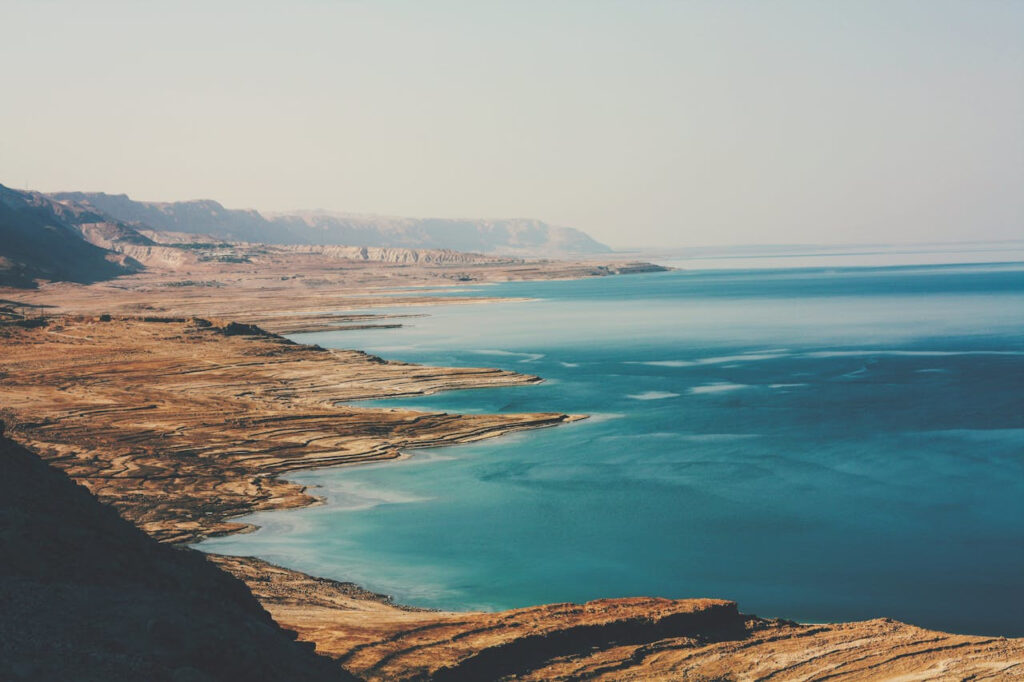
Figure 10: As in the case of the Jordan River, if a system is not actively linked with the total environment around it, it stops to function.
The efficiency of natural cycles has been significantly impacted by overproduction and various forms of pollution. When excessive pressure is placed on the natural system, it eventually ceases to function. Stagnation leads inevitably to death. The Dead Sea serves as a prime example. Water flows from the Golan Highlands through the River Jordan into the Dead Sea, which lacks an outlet. Over thousands of years, salts washed out of the soils have accumulated in this inland lake. No life thrives in the Dead Sea. It represents an ecosystem that has ceased to function due to the accumulation of dissolved substances, hindering circulation and the support of natural life. In nature, nothing can exist in isolation.
If a system is not actively connected to the broader environment, it will perish. Everything is inherently interconnected and interdependent for survival, functioning dynamically within the ecosystem.
It is evident that natural systems function harmoniously to sustain life on Earth. However, it is also evident that humans degrade the quality of our natural resources by disrupting the natural rhythm of nature. This disruption occurs when we, for example:
- * Mass-produce materials such as plastic and nuclear waste, which cannot be naturally recycled.
- * Continuously increase our reliance on fossil fuels, leading to air pollution and hindering the gaseous cycle responsible for purifying water vapor from impurities.
- * Overload rivers and estuaries with organic waste to the extent that marshes and reeds, which act as natural water purifiers, become ineffective.
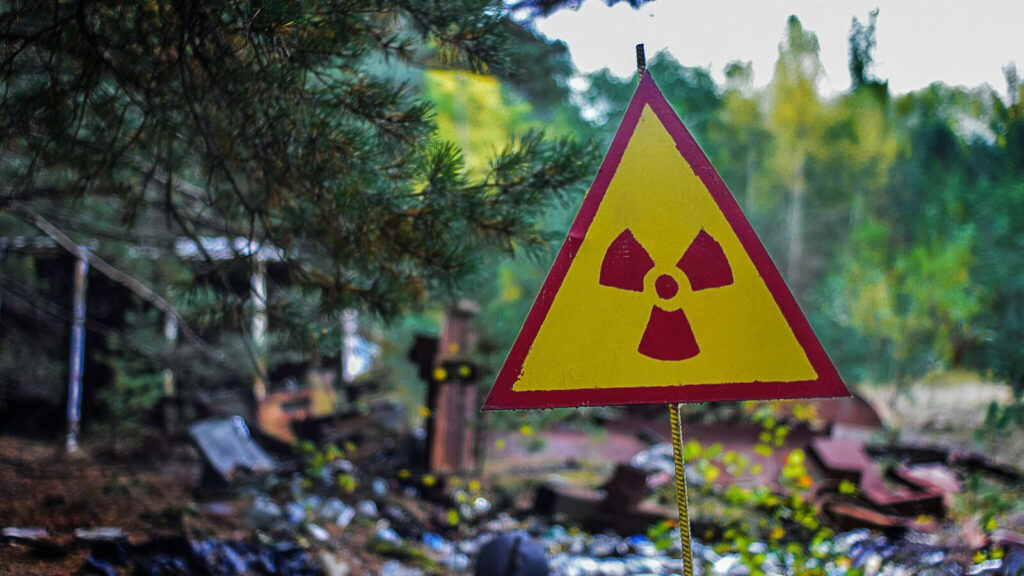
Figure 11: Until we have found a safe method to recycle or neutralize nuclear waste, we are jeopardizing the lives of future generations.
It is imperative to acknowledge that due to population explosions and escalating consumer demands, human activities are interfering with and consequently exerting increasing pressure on ecosystem cycles. Various mining activities have led to the exposure of Earth’s buried rocks to weathering and erosion, hastening the movement of elements in the global sedimentary cycle and potentially obstructing the system’s circulation.
The extraction of coal and oil for energy purposes is emitting carbon dioxide into the atmosphere at a rate approximately seventy times faster than natural processes would dictate, thereby impacting the gaseous cycle.
Humans possess significant capabilities to accelerate the movement of materials within both sedimentary and biological cycles. Disruption in any of these cycles could have catastrophic repercussions on our livelihoods.
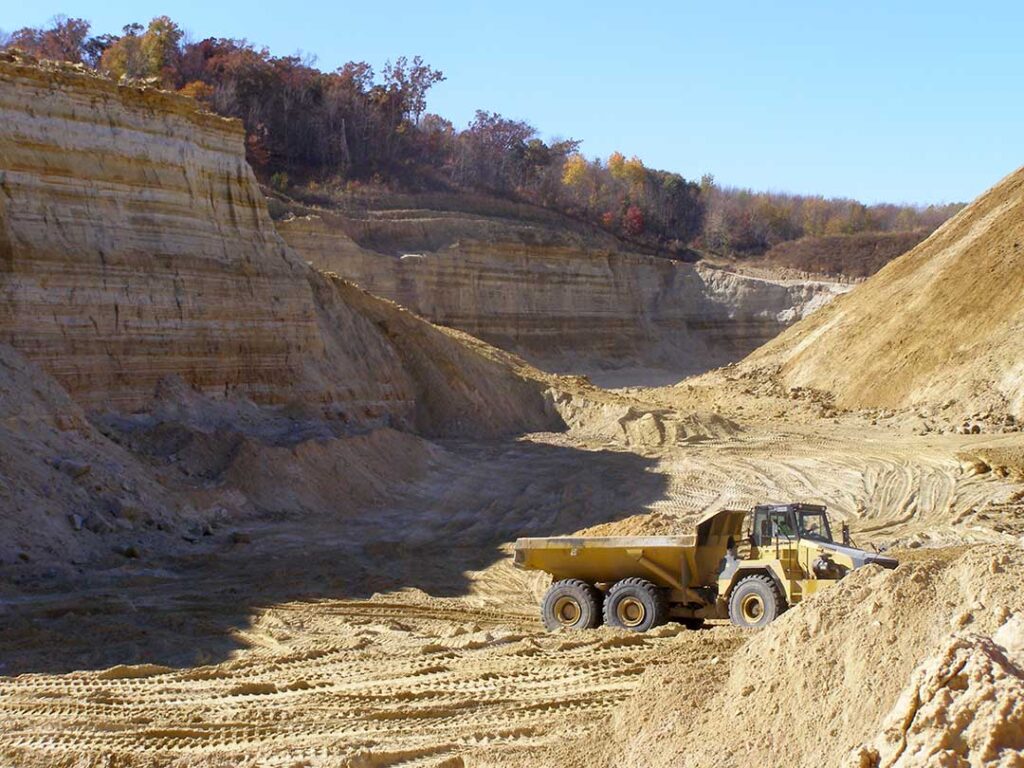
Figure 12: Due to activities such as mining we have accelerated the movement of sediments through the natural system.
The depletion of the world’s forests will significantly impede, or even halt, the cycling of CO2. This could not only lead to global temperatures rising dramatically, but humanity itself could ultimately face suffocation. It is imperative for us to reconsider our connection with nature. Without discovering and adopting sustainable approaches to production, agriculture, waste management, transportation, and other essential aspects, our reservoir of resources will continue to diminish, exerting intolerable strain on a growing global population. Urgent adherence to the fundamental laws of nature is imperative across all land use to guarantee sustainability.